May 10th, 2025
Can Life be based on Silicon instead of Carbon?
Alternative Biochemistries
Since around 1900, there have been considerations as to whether living organisms could also use other elements as building blocks instead of carbon. Life forms based on silicon, boron, nitrogen or sulphur, which can also form stable macromolecules under certain conditions, have been proposed as alternatives. Silicon-based life, in particular, has been the subject of serious discussion and has also been taken up in science fiction. Of the possible alternatives, silicon is still the most similar to carbon. But what about silicon from a chemical point of view? Could life actually exist on the basis of silicon?
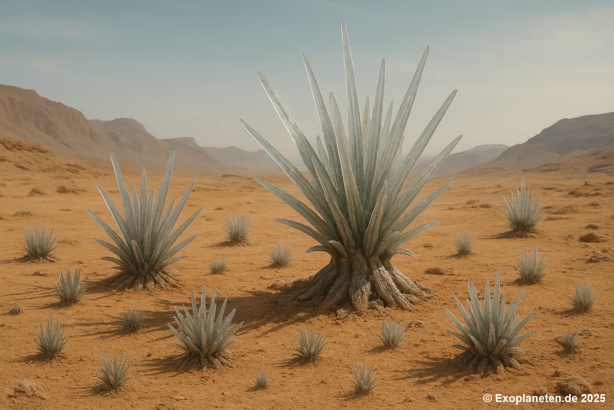
Basic requirements
Two things are particularly important for the chemistry of life. The ‘scaffold’, whether it is made of carbon or silicon, must be stable in a certain range of environmental conditions and should not dissolve immediately on contact with water or another natural solvent, for example. At the same time, the bonds must not be too strong so that a rearrangement or new formation of bonds is possible with comparatively moderate energy expenditure. All life processes are based on the formation and dissolution of various types of interatomic and intermolecular bonds. If the bonds are too difficult to break, life processes come to an end. If the bonds break too easily, everything flies apart and the organism dissolves or burns.
In addition to the framework, the largest possible number of functional groups is necessary. A pure hydrocarbon backbone is hardly reactive at all. Only functional groups enable controlled biochemistry. Frequently encountered functional groups are, for example, alcohols, ketones, phosphates, amines, ethers, esters and many others. The properties of the functional groups are often more important than the details of the framework. For example, the hydrocarbons methane, ethane and propane are gaseous at room temperature. However, the addition of the functional alcohol group (-OH) makes them liquids that can mix with water and are also open to completely different reactions than pure hydrocarbons.
Atoms are everything
In order to compare carbon and silicon in this respect, we need to look at three important variables: The atomic orbitals, electronegativity and the strength of the bond to other important elements.
In terms of atomic orbitals, silicon is very similar to carbon. Both elements have two electrons in the s orbital and two electrons in the p orbitals in their outer shell. To achieve the stable noble gas configuration, both elements can either donate four electrons or accept four electrons. In total, there is the possibility of four bonds, which are arranged in space like the corners of a three-sided pyramid (this shape is called a tetrahedron). In addition, silicon can also offer other atoms the d orbitals below this for bonding. Although d orbitals are not occupied in silicon, they are still available as empty orbitals for bonding. This explains the 5- or 6-bond nature of some silicon compounds. For organic chemistry, which requires the greatest possible variety of structures, this would not necessarily be a hindrance.
Electronegativity is a quantity that can be used to describe the attraction of atomic nuclei to electrons within molecules. Of all the chemical elements, fluorine has the highest electronegativity (3.98 on the Pauling scale), which means that fluorine attracts electrons to itself most strongly in a compound. The alkali metals, on the other hand, have electronegativity values between 0.7 and 0.98 and therefore have the lowest tendency to bind electrons – on the contrary, the alkali metals give up their only outer s-electron very readily, which is why they are very reactive. Carbon and silicon both have medium electronegativities: carbon 2.55 and silicon 1.9.
Chemistry of Methane and Silane
An interesting difference becomes clear when you look at the simplest compound of carbon and silicon with hydrogen. The gas methane (CH4) is the simplest hydrocarbon and as such is the basic unit of the higher hydrocarbons and the biochemically interesting compounds. The corresponding compound of silicon and hydrogen is silane (SiH4), which is also gaseous. The electronegativity of hydrogen (2.2) is now exactly between that of carbon and silicon. While the carbon in the methane molecule pulls the electrons towards itself on average and leaves the four hydrogen atoms with an electron deficit and a slightly positive partial charge, it is exactly the opposite way in silane. There, the four hydrogen molecules pull more strongly on the electrons, which gives them a slightly negative partial charge and the silicon atom a slight electron deficit and a positive partial charge.
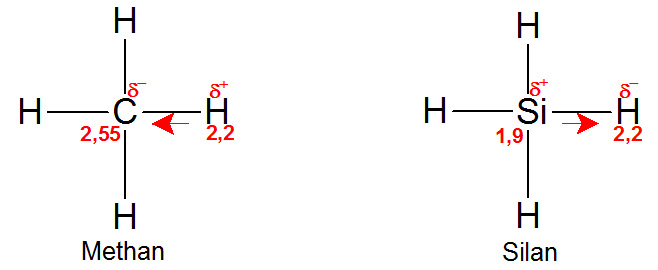
Chemically, this is very significant. In a mixture with normal air, both gases are essentially surrounded by the inert nitrogen and the reactive and at the same time very electronegative oxygen (electronegativity: 3.44). Methane burns with oxygen to form water and carbon dioxide, but requires activation energy, e.g. in the form of a spark. Without such an ignition, the electron-withdrawing oxygen molecules mainly come into contact with the electron-poor hydrogen atoms of methane – there is ‘nothing for the oxygen to gain’, the mixture remains stable for the time being. Only with the help of a spark can oxygen break the bond between carbon and hydrogen and react with both.
The exact opposite happens with a mixture of oxygen and silane. Here the oxygen is in contact with electron-rich hydrogen atoms. Together with the somewhat weaker silicon-hydrogen bond, this is enough to start the reaction on its own: oxygen breaks the bond between the silicon and the hydrogen, reacts with hydrogen to form water and with the silicon to form silicon dioxide (SiO2, glass). Silane is pyrophoric, which means that it ignites when it comes into contact with oxygen.
Within longer-chain molecules, the bond between two silicon atoms is about a quarter weaker than between two carbon atoms. This is a consequence of the larger radius of the silicon atom: the bonding electrons are further away from the positive charge of the atomic nucleus, so the attractive forces are lower. At the same time, the bond between silicon and oxygen is extremely strong and the two elements are very difficult to separate again.
Higher alkanes and silanes
Longer-chain hydrocarbons exist at room temperature as liquids or solids. Liquid hexane is one possible form, candle wax (paraffins) another. Hexane is lighter than water and floats on it, but does not react with water. Even a piece of candle wax will not change in water. For longer-chain silanes, however, this only applies in the absence of oxygen. Without oxygen, silanes can be stored stably on salty, aqueous solutions. However, if oxygen is added, this promotes the decomposition of the silanes. In alkaline solutions, the reaction takes place even faster with the formation of hydrogen and silicon hydroxide (Si(OH)4).
Higher bonds
We could compare the ability to form double and triple bonds and realise that carbon has many more possibilities here than silicon. Both types of bond are of enormous importance for biochemistry because they enable additional structural and chemical diversity. However, we can already draw an important conclusion at this point and consider the question of how likely the natural formation of silicon biochemistry is in comparison to carbon biochemistry. Carbon forms thousands of compounds that remain stable long enough in the presence of oxygen or in aqueous solution to support a functioning biochemistry; many carbon compounds have also been detected in interstellar space. In the event of combustion, gaseous carbon dioxide is produced, which is soluble in water and thus accessible to further chemical reactions. However, the analogue compounds of silicon are unstable in the presence of oxygen and water. One of the decomposition products is glass, which can hardly react chemically.
Oxygen as the big killer of Silicoids
Oxygen is the third most common element in the gas nebulae from which stars and planets are formed. Within the Earth’s crust, it is even the second most common element. Water is also omnipresent in the universe and has already been detected in quasars, protoplanetary nebulae and exoplanets. Even on a planet without free, gaseous oxygen and without water, oxygen would still be bound in the minerals. The most important components of the Earth’s mantle are forms of magnesium oxide and aluminium oxide. The probability that the precursors of silicon biochemistry on other planets also end up in the form of unreactive glass is therefore very high. Life on the basis of silicon is therefore likely to remain in the realm of science fiction. Carbon is a much more suitable element for this function.
References
Clayden, J., Greeves, N., & Warren, S. G. (2013). Organic Chemistry. Springer Spectrum.
Rate this article: